Students & Educators —Menu
- Educational Resources
- Educators & Faculty
- Standards & Guidelines
- Periodic Table
- Adventures in Chemistry
- Landmarks Directory
- Frontiers of Knowledge
- Medical Miracles
- Industrial Advances
- Consumer Products
- Cradles of Chemistry
- Nomination Process
- Science Outreach
- Publications
- ACS Student Communities
- LEADS Conference
- ChemMatters
- Chemistry Outreach Activities
- National Chemistry Week
- U.S. National Chemistry Olympiad
- You are here:
- American Chemical Society
- Students & Educators
- Explore Chemistry
- Chemical Landmarks
- Antoine Laurent Lavoisier

The Chemical Revolution of Antoine-Laurent Lavoisier
International historic chemical landmark.
Dedicated June 8, 1999, at the Académie des Sciences de l’Institut de France in Paris, France.
Commemorative Booklet (PDF)
En español: La revolución química de Antoine-Laurent Lavoisier
Antoine-Laurent Lavoisier forever changed the practice and concepts of chemistry by forging a new series of laboratory analyses that would bring order to the chaotic centuries of Greek philosophy and medieval alchemy. Lavoisier’s work in framing the principles of modern chemistry led future generations to regard him as a founder of the science.
Beliefs in Chemistry at Lavoisier’s Time
Combustion and the attack on phlogiston, a new chemistry emerges, the life of antoine-laurent lavoisier (1743-1794), further reading, landmark designation and acknowledgments, cite this page.
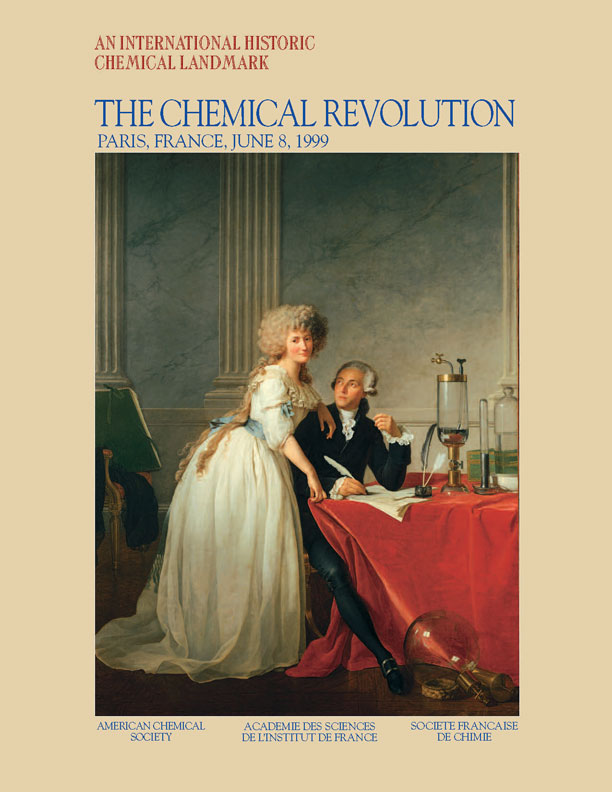
When the 17-year-old Lavoisier left Mazarin College in Paris in 1761, chemistry hardly could be called a true science. Unlike physics, which had come of age through the work of Isaac Newton a century earlier, chemistry was still mired in the legacy of the Greek philosophers. The four elements of Aristotle — earth, air, fire, and water — had been slowly modified by the medieval alchemists, who added their own arcane language and symbolism.
Thrown into this mix was the concept of phlogiston. Developed by the German scientist Georg Ernst Stahl early in the 18th century, phlogiston was a dominant chemical concept of the time because it seemed to explain so much in a simple fashion. Stahl believed that every combustible substance contained a universal component of fire, which he named phlogiston, from the Greek word for inflammable. Because a combustible substance such as charcoal lost weight when it burned, Stahl reasoned that this change was due to the loss of its phlogiston component to the air.
It followed that the less residue a substance left after burning, the greater its phlogiston content. Turning from organic substances to metals, Stahl knew that a metal calx (known today as an oxide) heated with charcoal formed the original metal. He proposed that the phlogiston of the charcoal had united with the calx. Therefore, metals, which were thought to contain phlogiston, were also classified as combustibles.
The difficulty with this scheme was the reverse reaction. When metals were strongly heated in air, the resulting calx weighed more than the original metal, not less, as would be expected if the lead had lost the phlogiston component. This inconsistency caused some phlogistonists to suggest that phlogiston might even have a negative weight. Lavoisier was introduced to phlogiston by Guillaume Franåois Rouelle, whose lectures he attended while pursuing a law degree. By 1772, having abandoned law to pursue a career in science, Lavoisier turned his curiosity to the study of combustion.
Back to top

The importance of the end in view prompted me to undertake all this work, which seemed to me destined to bring about a revolution in…chemistry. An immense series of experiments remains to be made."
— Antoine-Laurent Lavoisier, February 20, 1773
In experiments with phosphorus and sulfur, both of which burned readily, Lavoisier showed that they gained weight by combining with air. With lead calx, he was able to capture a large amount of air that was liberated when the calx was heated. To a suspicious Lavoisier, these results were not explained by phlogiston.
Although Lavoisier now realized that combustion actually involved air, the exact composition of air at that time was not clearly understood. In August 1774, the eminent English natural philosopher Joseph Priestley met with Lavoisier in Paris. He described how he had recently heated mercury calx (a red powder) and collected a gas in which a candle burned vigorously. Priestley believed his "pure air" enhanced respiration and caused candles to burn longer because it was free of phlogiston. For this reason, he called the gas that he obtained from decomposing mercury calx “dephlogisticated air.”
In Paris, the intrigued Lavoisier repeated Priestley's experiment with mercury and other metal calces. He eventually concluded that common air was not a simple substance. Instead, he argued, there were two components: one that combined with the metal and supported respiration and the other an asphyxiant that did not support either combustion or respiration. By 1777, Lavoisier was ready to propose a new theory of combustion that excluded phlogiston. Combustion, he said, was the reaction of a metal or an organic substance with that part of common air he termed "eminently respirable." Two years later, he announced to the Royal Academy of Sciences in Paris that he found that most acids contained this breathable air. Lavoisier called it oxygène, from the two Greek words for acid generator.
Lavoisier began his full-scale attack on phlogiston in 1783, claiming that "Stahl's phlogiston is imaginary." Calling phlogiston "a veritable Proteus that changes its form every instant," Lavoisier asserted that it was time "to lead chemistry back to a stricter way of thinking" and "to distinguish what is fact and observation from what is system and hypothesis." As a starting point, he offered his theory of combustion, in which oxygen now played the central role.
Early Beliefs about Phlogiston
In the mid-18th century, the most pressing issue in chemistry and physics was to determine what exactly happens when something burns. The prevailing theory was that flammable materials contained a substance called “phlogiston” (from the Greek word for burn) that was released during combustion.
The theory held that when a candle burned, for example, phlogiston was transferred from it to the surrounding air. When the air became saturated with phlogiston and could contain no more, the flame went out. Breathing, too, was a way to remove phlogiston from a body. A typical test for the presence of phlogiston was to place a mouse in a container and measure how long it lived. When the air in the container could accept no more phlogiston, the mouse would die.
Antoine Lavoisier disproved the existence of phlogiston and helped to form the basis of modern chemistry using Joseph Priestley’s discovery of oxygen.
In 1766, Englishman Henry Cavendish isolated a gas that he called "inflammable air" because it burned readily. Priestley noted that when inflammable air and common air were ignited with a spark in a closed vessel, a small amount of "dew" formed on the glass walls. When Cavendish repeated the experiment, he found that the dew was actually water. Cavendish explained the results in terms of phlogiston and assumed the water was present in each of the two airs before ignition.
For Lavoisier, combustion meant combining with oxygen; however, until he could explain the combustion of inflammable air, some would still doubt his new chemistry. In June 1783, Lavoisier reacted oxygen with inflammable air, obtaining "water in a very pure state." He correctly concluded that water was not an element but a compound of oxygen and inflammable air, or hydrogen as it is now known. To support his claim, Lavoisier decomposed water into oxygen and inflammable air. Now that the composition of water was known, the last objection to discarding phlogiston could be eliminated.
To Lavoisier, it was time "to rid chemistry of every kind of impediment that delays its advance" with a reform that included a new language. Louis Bernard Guyton de Morveau, Claude Louis Berthollet, Antoine Franåois Fourcroy, and Lavoisier adopted the long-neglected idea of an element as originally proposed by Robert Boyle more than a century earlier. They retained the names from the past of many simple substances, or elements. But when an element combined with another element, the compound's name now reflected something about its chemical composition. For example, a calx was the combination of a metal and oxygen; therefore, zinc calx became zinc oxide. Lavoisier and his colleagues predicted that if the new system was "undertaken upon sound principles...it will naturally adapt itself to future discoveries." Withstanding the test of time, the basic system is still in use today.
Lavoisier's new system of chemistry was laid out for everyone to see in the Traité élémentaire de Chimie (Elements of Chemistry), published in Paris in 1789. As a textbook, the Traité incorporated the foundations of modern chemistry. It spelled out the influence of heat on chemical reactions, the nature of gases, the reactions of acids and bases to form salts, and the apparatus used to perform chemical experiments. For the first time, the Law of the Conservation of Mass was defined, with Lavoisier asserting that "... in every operation an equal quantity of matter exists both before and after the operation." Perhaps the most striking feature of the Traité was its "Table of Simple Substances," the first modern listing of the then-known elements.
Lavoisier did not expect his ideas to be adopted at once, because those who believed in phlogiston would "adopt new ideas only with difficulty." Lavoisier put his faith in the younger generation who would be more open to new concepts. Two years later, in 1791, the results were obvious. "All young chemists," he mused, "adopt the theory, and from that I conclude that the revolution in chemistry has come to pass." His legacy endures more than 200 years later.
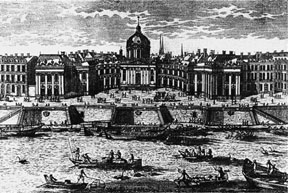
"Lavoisier was a Parisian through and through and a child of the enlightenment," wrote biographer Henry Guerlac. The son of Jean-Antoine and Émilie Punctis Lavoisier, he entered Mazarin College when he was 11. There, he received a sound training in the arts and classics and an exposure to science that was the best in Paris. Forgoing his baccalaureate of arts degree, Lavoisier yielded to the influence of his father and studied law, receiving a law degree in 1763. But his interest in science prevailed, kindled by the geologist Jean-Étienne Guettard, whom he met at Mazarin. After graduation, he began a long collaboration with Guettard on a geological survey of France.
Lavoisier showed an early inclination for quantitative measurements and soon began applying his interest in chemistry to the analysis of geological samples, especially gypsum. Because of his flair for careful analyses and his prodigious output, he was elected to the Academy of Sciences at the age of 25. At the same time, Lavoisier used part of the fortune he had inherited from his mother to buy a share in the Ferme Générale, a private group that collected various taxes for the government. This fateful decision would later cost him his life at the height of his intellectual powers.
He married Marie Anne Pierrette Paulze on Dec. 16, 1771; he was 28, she was 14. "The marriage was a happy one," according to Lavoisier biographer Douglas McKie. "Mme Lavoisier was possessed of a high intelligence; she took a great interest in her husband's scientific work and rapidly equipped herself to share in his labors. Later, she helped him in the laboratory and drew sketches of his experiments. She made many of the entries in his laboratory notebooks. She learned English and translated a number of scientific memoirs into French."
Lavoisier became further involved in public life in 1775, when he was appointed one of four commissioners of the Gunpowder Commission, charged with reforming and improving the production of gunpowder. Lavoisier moved his residence and laboratory to the arsenal in Paris, where for almost 20 years it drew many distinguished visitors. He devoted several hours every day and one full day a week to experiments in his laboratory. According to his wife: "It was for him a day of happiness; some friends who shared his views and some young men proud to be admitted to the honor of collaborating in his experiments assembled in the morning in the laboratory. There they lunched; there they debated... It was there that you could have heard this man with his precise mind, his clear intelligence, his high genius, the loftiness of his philosophical principles illuminating his conversation."
Ironically, Lavoisier, the ardent and zealous chemical revolutionary, was caught in the web of intrigue of a political revolution. The TraitÉ was published in 1789, the same year as the storming of the Bastille. A year later, Lavoisier complained that "the state of public affairs in France...has temporarily retarded the progress of science and distracted scientists from the work that is most precious to them."
Lavoisier, however, could not escape the wrath of Jean-Paul Marat, the adamant revolutionary who began publicly denouncing him in January 1791. During the Reign of Terror, arrest orders were issued for all of the Ferme Générale, including Lavoisier. On the morning of May 8, 1794, he was tried and convicted by the Revolutionary Tribunal as a principal in the "conspiracy against the people of France." He was sent to the guillotine that afternoon. The next day, his friend, the French mathematician Joseph-Louis Lagrange, remarked that "it took them only an instant to cut off that head, and a hundred years may not produce another like it."
- Société Française de Chimie (Chemical Society of France)
- Antione-Laurent Lavoisier (Chemical Heritage Foundation)
Landmark Designation
The American Chemical Society and the Société Française de Chimie designated Antoine-Laurent Lavoisier: The Chemical Revolution as an International Historic Chemical Landmark at the Royal Academy of Sciences in Paris on June 8, 1999. The English version of the text of the plaque presented to the Académie des Sciences de l'Institut de France reads:
In these buildings, then "Collège Mazarin" or "des Quatre-Nations," Antoine-Laurent Lavoisier (1743-1794) studied from 1754 to 1761. He was elected to the Royal Academy of Sciences in 1768, where he presented his important studies on oxygen in chemistry. These began with a "pli cacheté" of Nov. 2, 1772, and, after he experimentally proved the chemical composition of water by the quantitative method, culminated in his abandoning of the phlogistic theory in 1785. In 1787, he proposed the principles of a new M éthode de Nomenclature Chimique , in collaboration with the chemists Guyton de Morveau, Berthollet, and Fourcroy and with the help of the mathematicians Monge and Laplace. The publication of his Traité Elémentaire de Chimie two years later convinced French and foreign chemists of his theories. His papers, stored in the Archives of the Academy of Sciences, bear witness to the conception and maturing of his revolutionary ideas, which are at the foundations of modern chemistry.
Acknowledgments
Adapted for the internet from “The Chemical Revolution," produced by the National Historic Chemical Landmarks program of the American Chemical Society in 1999.
American Chemical Society International Historic Chemical Landmarks. Antoine-Laurent Lavoisier: The Chemical Revolution. http://www.acs.org/content/acs/en/education/whatischemistry/landmarks/lavoisier.html (accessed Month Day, Year).
Back to National Historic Chemical Landmarks Main Page .
Learn more: About the Landmarks Program .
Take action: Nominate a Landmark and Contact the NHCL Coordinator .

Accept & Close The ACS takes your privacy seriously as it relates to cookies. We use cookies to remember users, better understand ways to serve them, improve our value proposition, and optimize their experience. Learn more about managing your cookies at Cookies Policy .
1155 Sixteenth Street, NW, Washington, DC 20036, USA | service@acs.org | 1-800-333-9511 (US and Canada) | 614-447-3776 (outside North America)
- Terms of Use
- Accessibility
Copyright © 2024 American Chemical Society
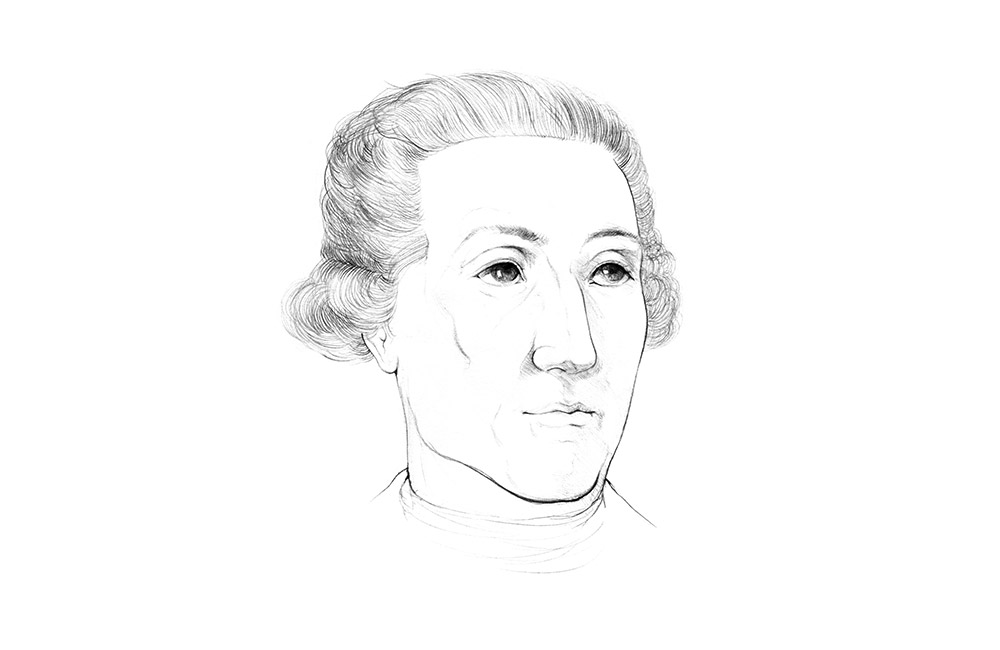
Antoine Lavoisier
Lavoisier was born on August 26th, 1743 in Paris, France. Lavoisier discovered no new substances nor new chemical reactions, but his greatest achievement was the establishment of a new theoretical framework within which others’ experimental results could be properly explained. Lavoisier stressed on the importance of accurate measurements, and used quantitative experiments to test his ideas. He started a chemical revolution which put an end to the once popular phlogiston theory. In the center of this revolution was the elucidation of oxygen’s function in combustion. During French Revolution, Lavoisier, as a nobleman, was accused of several crimes, and was eventually guillotined at age of 50 on May 8th, 1794 in Paris, France. Lavoisier’s main contributions to science are:
Through quantitative experiments, elucidated the role of oxygen in combustion and metal calcination, putting an end to phlogiston theory.
Presented modern concepts of chemical elements, invalidated the Greek four element theory.
Invented modern nomenclature for chemical substances to replace old empirical one.
Authored the famous textbook Traité Élémentaire de Chimie , popularized the new chemical system.
Lavoisier's Instruments
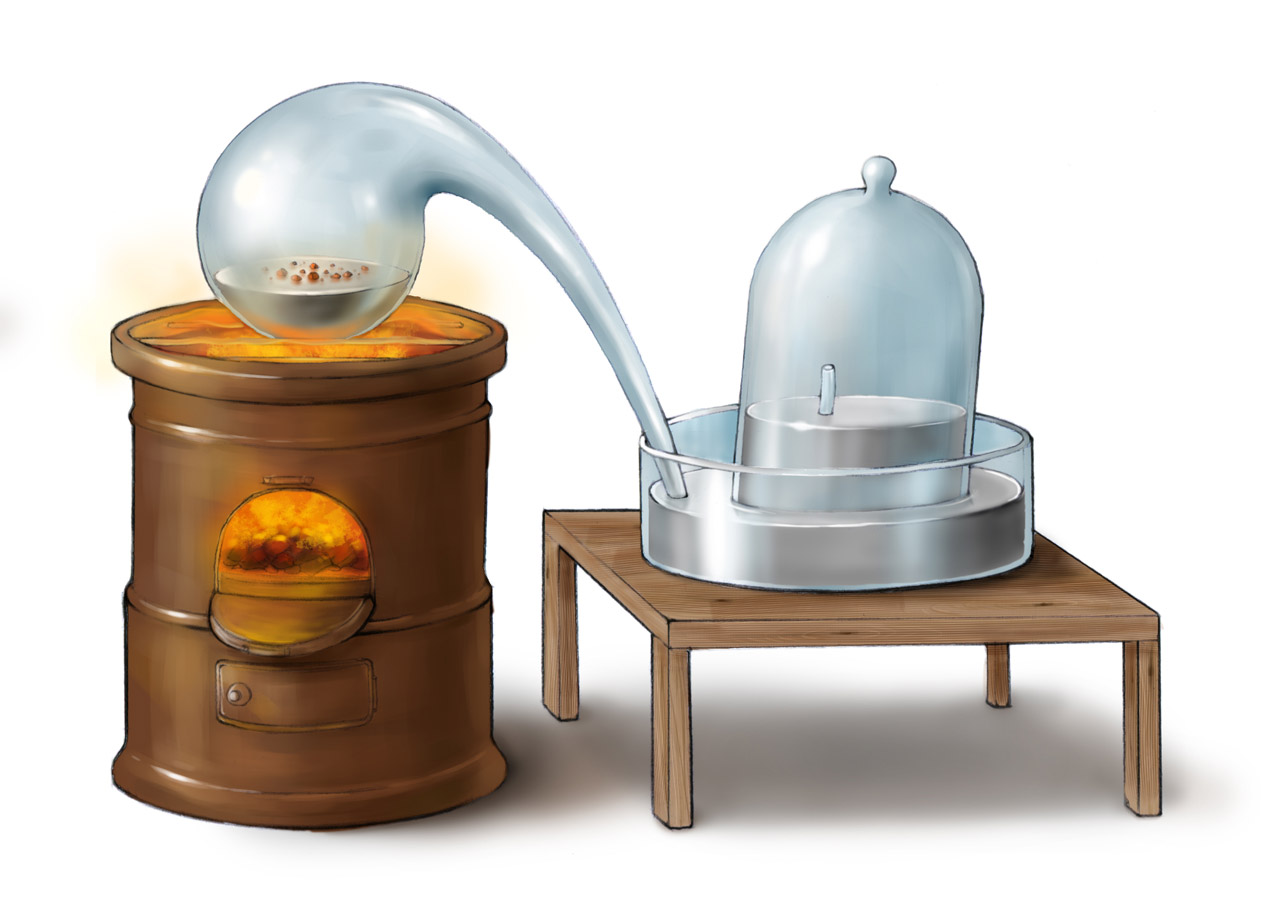
Above shows Lavoisier’s apparatus for studying mercury oxidation in closed environment described in his Traité Élémentaire de Chimie published in 1789 (a photorealistic CG reconstruction can be found here ). The system contained mercury in the resort and normal air sealed by a bell jar placed in the mercury reservoir. After heating the mercury in the resort for several days, red mercury oxide was observed on mercury surface. The mercury level inside the bell jar rose up because the consumption of oxygen. When the amount of mercury oxide no longer increased, the heating was terminated and the amount of gas volume decrease was measured. Lavoisier found that the gas loss was 16% of the total volume. The the mercury oxide was removed and heated again, the volume of oxygen generated was measured. It was found that the volume was the same as the 16% volume loss. The oxygen percentage (16%) was not accurate, which could be due to not all oxygen react with mercury. From this experiment, we can feel Lavoisier’s emphasize on the conservation of mass in his experiment design.
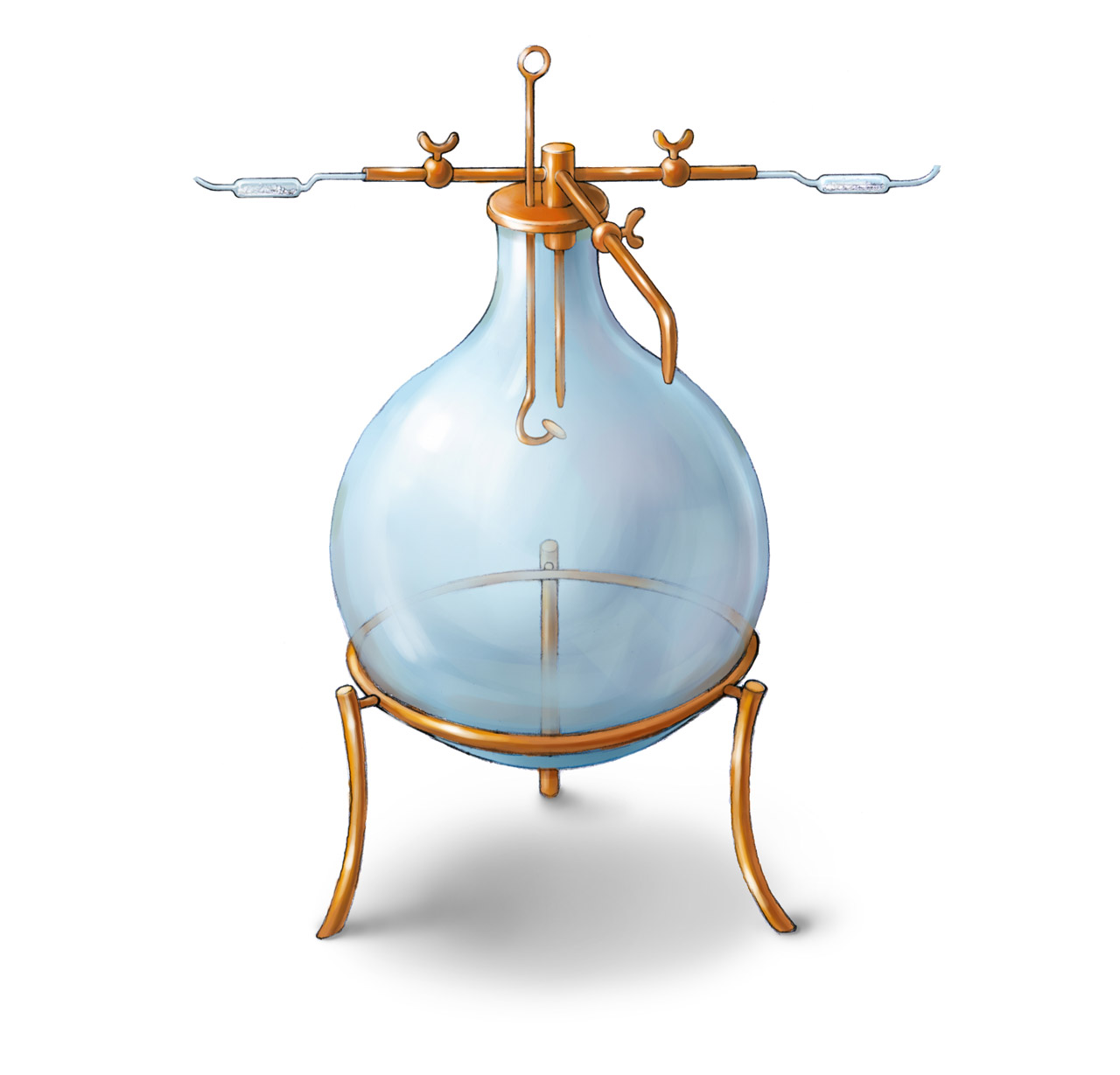
Above is Lavoisier’s apparatus for determine the composition of water through burning hydrogen in oxygen, described in his Traité Élémentaire de Chimie published in 1789. Before the reaction, the air inside the apparatus was removed by a vacuum pump. Then oxygen was let in through the right pipe and hydrogen through the left pipe. As soon as hydrogen entered the contrainer, an electric spark was initiated to ignite the combustion. The flux ration of oxygen and hydrogen was 1:2 and combustion was maintained for a long time. The water generated was collected in the bottom of the container. After the experiment, a crucial check was that the total mass of oxygen and hydrogen consumed should equal the water produced. Through this experiment, Lavoisier proved that water was not an element, but a compound made of hydrogen and oxygen, with a weight percentage of 15% and 85%, respectively. We now know that the correct percentage should be 11% for hydrogen and 89% for oxygen. In terms of accuracy, Lavoisier’s experiment was not as good as Cavendish’s.
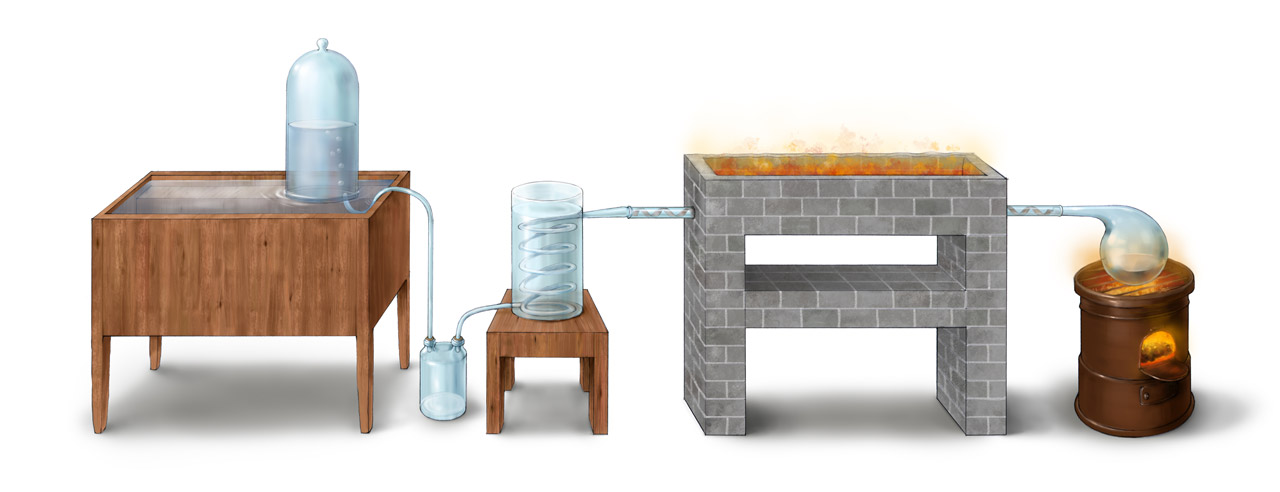
Above is Lavoisier’s apparatus for studying the reaction between water and iron at high temperature, described in his Traité Élémentaire de Chimie published in 1789. The goal of this experiment was to determine the composition of water. Through heating the distilled water inside the right retort, water steam was transported to the high-temperature glass tube, reacting with the spiral shaped ion sheet inside. The reaction between water and iron generated black iron oxide (Fe3O4) and hydrogen. The unreacted water steam passing the long tube was condensed in the condenser and collected in a bottle. Hydrogen was collected with the bell jar sitting on the platform of the water trough and its mass measured. The mass increase of the iron sheet came from the oxygen in water. Lavoisier carefully checked that the mass of reacted water (the mass loss of the retort subtracted from the mass increase of the collecting bottle) equaled the total mass of hydrogen collected in the bell jar and oxygen calculated from iron weight increase. Through this complicated setup, Lavoisier again found the hydrogen and oxygen weight percentage of water is 15% and 85%, respectively. This result was again not as accurate as Cavendish’s.
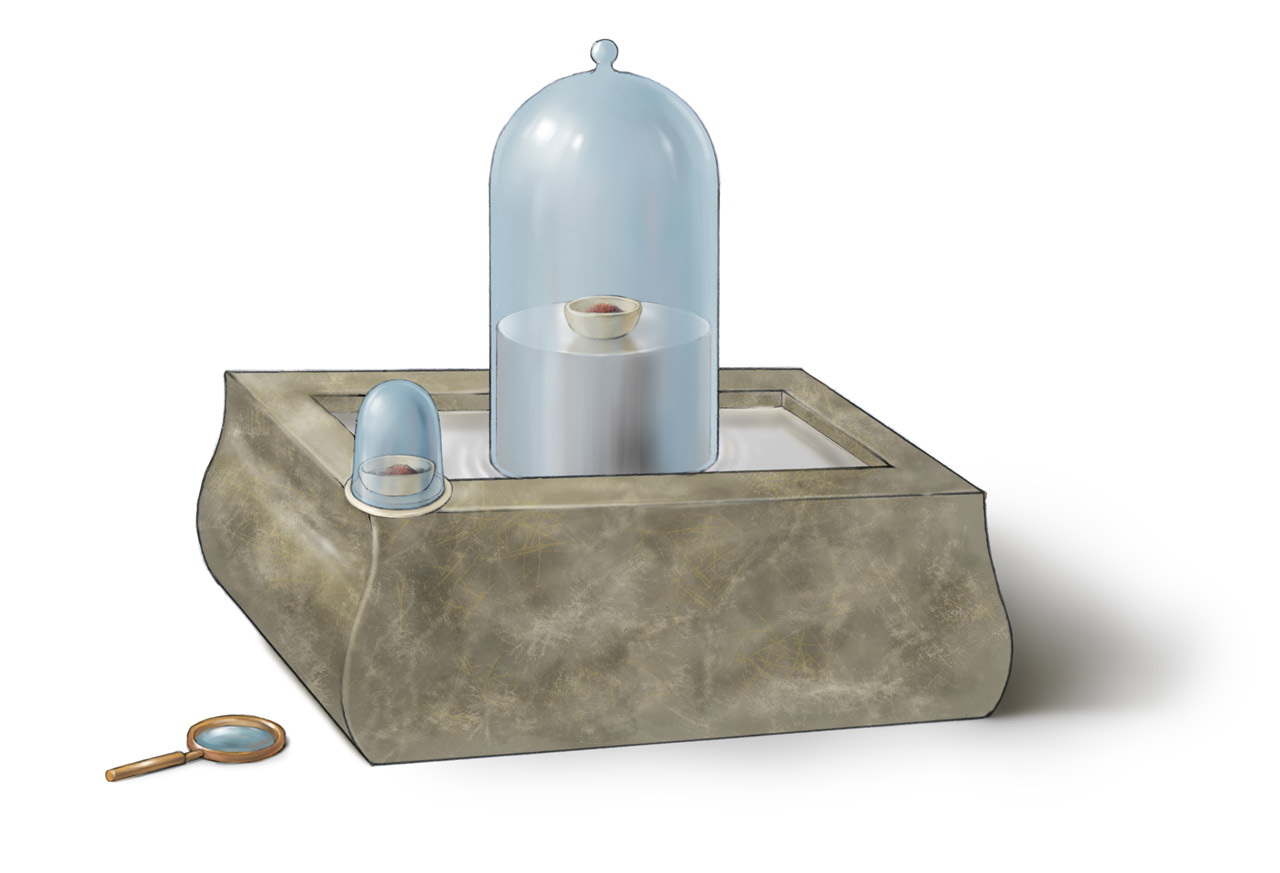
Above is Lavoisier’s apparatus for studying red phosphorus combustion inside oxygen, described in his Traité Élémentaire de Chimie published in 1789 (a photorealistic CG reconstruction can be found here . The bell jar over the mercury reservoir contained oxygen. The red phosphorus was transferred into the bell jar through a small sealed container. The red phosphorus was ignited by a red-hot ion stick with a special shape or a burning glass. Lavoisier also used this apparatus to studied the combustion of other substances. In his book, he warned his readers not to use the apparatus to study volatile substance like alcohol, which was likely to explode. With this apparatus, Lavoisier could determine the amount of oxygen reacted with phosphorus. However, he could not determine the amount of phosphorus oxide produced in the reaction. As a result, he designed a new apparatus to make more accurate measurement.
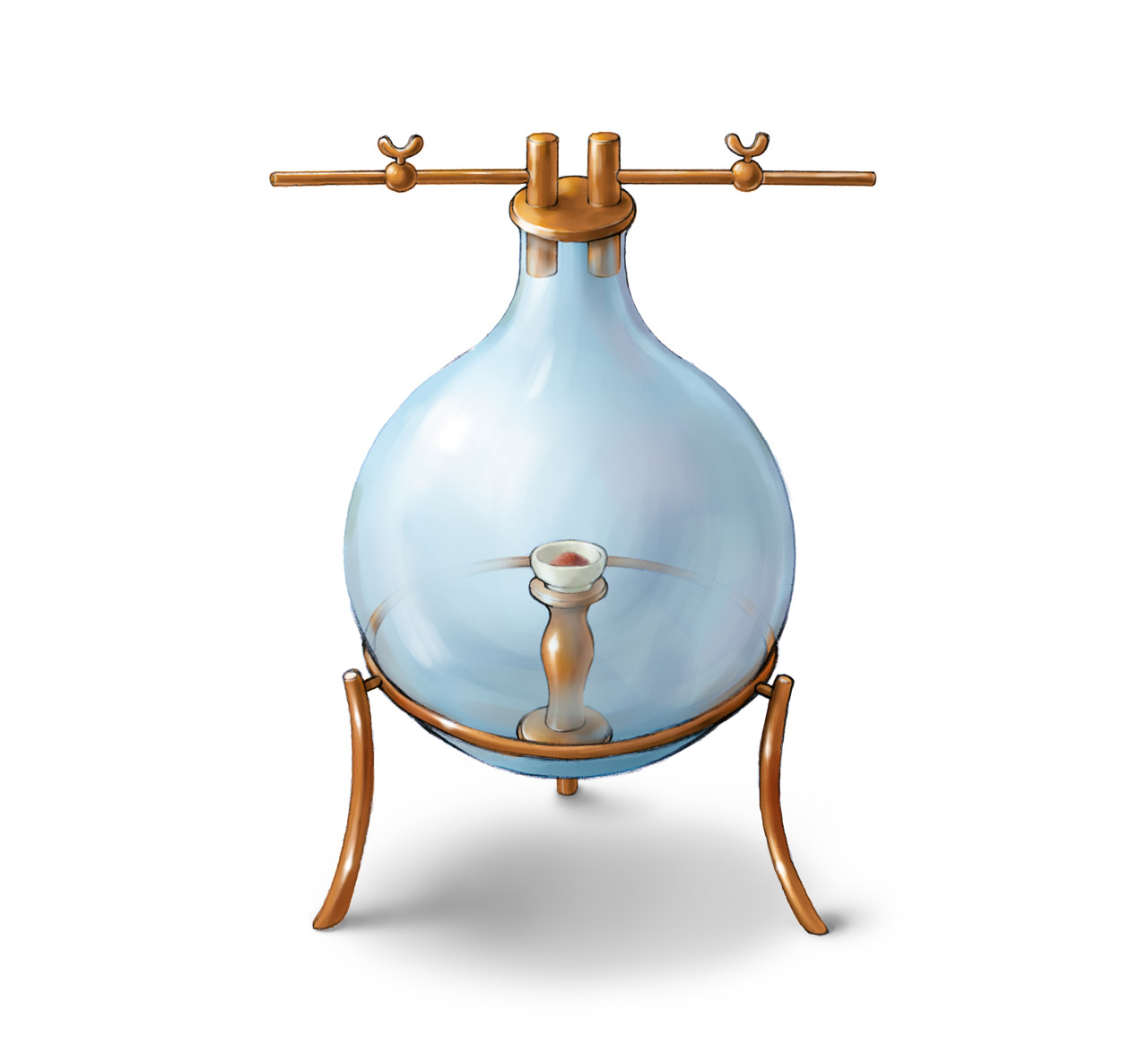
Above is Lavoisier’s another apparatus for studying red phosphorus combustion inside oxygen, described in his Traité Élémentaire de Chimie published in 1789. First he placed a small bow containing red phosphorus inside a glass globe, then sealed the opening. After the lute dried up, the whole apparatus was weighted. Then the air inside the globe was removed by a vacuum pump. Another through another tube, oxygen was introduced. A burning glass was used to ignite the red phosphorus and oxygen flow was maintained until all the phosphorus was burnt up. By weighting the whole apparatus at the end, the weight of the phosphorus oxide could be determined.
Other Chemists
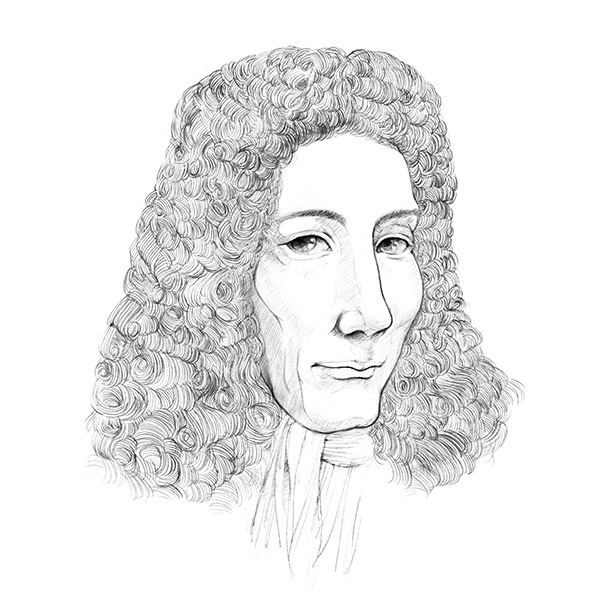
Antoine Lavoisier’s Theory of Combustion
Antoine Lavoisier conducting an experiment related combustion generated by amplified sun light.
On Nov 1, 1772, French chemist Antoine Lavoisier [ 1 ] reported in a note to the Secretary of the French Academy of Sciences about the role of “air” in the combustion process. It required five more years of experiments, before in 1777 , Lavoisier was ready to propose a new theory of combustion that excluded phlogiston ,[ 4 ] which according to the prevailing theories of the time was part of every matter and responsible for the combustion process.
“Here, then: a revolution [in science and chemistry] has taken place in an important part of human knowledge since your departure from Europe… I will consider this revolution to be well advanced and even completely accomplished if you range yourself with us. …After having brought you up to date on what is happening in chemistry, it would be well to speak to you about our political revolution. We regard it as done and without any possibility of return to the old order.” – Antoine Lavoisier, Letter to Benjamin Franklin (Feb 2, 1790)
Modern Chemistry started with Lavoisier
Modern chemistry started with Antoine Lavoisier , as we have already learned in a previous article about Lavoisier’s life and achievements [ 1 ]. Today, we focus on one particular subject, Lavoisier`s theory of combustion. During late 1772 Lavoisier turned his attention to the phenomenon of combustion, the topic on which he was to make his most significant contribution to science. In the times of Lavoisier, processes such as combustion and rusting , have not been related to oxidation like nowadays. In 1667 , German chemist Johann Joachim Becher [ 4 ] had published his so-called phlogiston theory. The theory attempted to explain all burning processes. in general, substances that burned in air were said to be rich in a substance called phlogiston . The fact that combustion soon ceased in an enclosed space was taken as clear-cut evidence that air had the capacity to absorb only a finite amount of phlogiston. When air had become completely phlogisticated it would no longer serve to support combustion of any material, nor would a metal heated in it yield a calx ; nor could phlogisticated air support life. Breathing was thought to take phlogiston out of the body. Thus, Becher described phlogiston as a process that explained combustion through a process that was opposite to that of oxygen.
Lavoisier’s Experiments
Antoine Lavoisier reported the results of his first experiments on combustion in a note to the Academy on 20 October 1772, in which he reported that when phosphorus burned, it combined with a large quantity of air to produce acid spirit of phosphorus, and that the phosphorus increased in weight on burning. In a second sealed note deposited with the Academy a few weeks later on November 1, 1772, Lavoisier extended his observations and conclusions to the burning of sulfur and went on to add that “ what is observed in the combustion of sulfur and phosphorus may well take place in the case of all substances that gain in weight by combustion and calcination: and I am persuaded that the increase in weight of metallic calces is due to the same cause .” Although Lavoisier now realized that combustion actually involved air, the exact composition of air at that time was not clearly understood.
Antoine Lavoisier’s phlogiston experiment. Engraving by Mme Lavoisier in the 1780s taken from Traité Élémentaire de Chimie
Exchange with Priestley
In the spring of 1774 Lavoisier carried out experiments on the calcination of tin and lead in sealed vessels which conclusively confirmed that the increase in weight of metals in combustion was due to combination with air. But the question remained about whether it was combination with common atmospheric air or with only a part of atmospheric air. In October the eminent English natural philosopher Joseph Priestley visited Paris [ 2 ], where he met Lavoisier and told him of the air which he had produced by heating the red calx of mercury with a burning glass and which had supported combustion with extreme vigor. Priestley at this time was unsure of the nature of this gas, but he felt that it was an especially pure form of common air.
Lavoisier’s Theory of Combustion
In Paris, the intrigued Lavoisier repeated Priestley’s experiment with mercury and other metal calces. He eventually concluded that common air was not a simple substance. Instead, he argued, there were two components: one that combined with the metal and supported respiration and the other an asphyxiant that did not support either combustion or respiration. By 1777 , Lavoisier was ready to propose a new theory of combustion that excluded phlogiston. Phlogiston remained the dominant theory until the 1780s when Lavoisier showed that combustion requires a gas that has mass (oxygen) and could be measured by means of weighing closed vessels. Lavoisier’s chemical research between 1772 and 1778 was largely concerned with developing his own new theory of combustion. In 1783 he read to the academy his famous paper entitled Réflexions sur le phlogistique (Reflections on Phlogiston), a full-scale attack on the current phlogiston theory of combustion.
To promote the breakthrough of his oxidation theory, Lavoisier organized a stage play in June 1789, which he performed at the Arsenal. On the stage appeared the flammable phlogiston, it was accused of serious crimes by Oxygène. The advocate, Professor Stahl, defended the phlogiston. Finally, phlogiston was sentenced to death by fire. Madame Lavoisier was the sacrificial priestess in this.
Related work and further reading:
- [1] Modern Chemistry started with Lavoisier , SciHi Blog
- [2] Joseph Priestley and the Discovery of Oxygen , SciHi Blog.
- [3] The Chemical Revolution of Antoine-Laurent Lavoisier , International Historic Chemical Landmark, Dedicated June 8, 1999, at the Académie des Sciences de l’Institut de France in Paris, France.
- [4] Johann Joachim Becher and the Phlogiston Theory of Combustion , SciHi Blog
- [5] Michael Hoepfner, Lecture 8 – Combustion Reactions (Jan. 26, 2018) , Michael Hoepfner @ youtube
- [6] Works by or about Antoine Lavoisier at Internet Archive
- [7] Oeuvres de Lavoisier (Works of Lavoisier) at Gallica BnF in six volumes.
- [8] Antoine Lavoisier at Wikidata
- [9] Antoine Lavoisier Timeline via Wikidata
Harald Sack
Related posts, frederick william twort and the bacteriophages, edward c. kendall and the adrenal cortex hormones, hans von euler-chelpin and the alcoholic fermentation of sugar, alexandre-émile béguyer de chancourtois and the order of the chemical elements, one comment.
Pingback: Whewell’s Gazette: Year 2, Vol. #17 | Whewell's Ghost
Leave a Reply Cancel reply
Your email address will not be published. Required fields are marked *
Further Projects
- February (28)
- January (30)
- December (30)
- November (29)
- October (31)
- September (30)
- August (30)
- January (31)
- December (31)
- November (30)
- August (31)
- February (29)
- February (19)
- January (18)
- October (29)
- September (29)
- February (5)
- January (5)
- December (14)
- November (9)
- October (13)
- September (6)
- August (13)
- December (3)
- November (5)
- October (1)
- September (3)
- November (2)
- September (2)
Legal Notice
- Privacy Statement
Lavoisier and the Discovery of Combustion

Lavoisier started by using mercury. When heated, mercury formed mercury oxide, in the form of small red balls. Lavoisier was interested in the composition of this red solid. Therefore he tried to reduce the mercury oxide: Lavoisier placed an ounce of mercury oxide and 48 grains of coal in a round-bottomed flask with the neck of the flask extending into a tube. This mixture was heated. The end of the tube from the flask
was placed into a bowl of water with the opening of the tube directly underneath a semi-submerged bell jar. As gas is produced in the flask, the gas travels through the tube and into the bell jar, thus displacing the water in the bell jar. The water level at the start and end of the experiment was measured.
According to his scientific observations, a colourless gas was released. The most important property of this gas was that a candle extinguished in its presence almost instantaneously. Also, it reacted with Lime Water to form a cloudy solution. These properties were characteristic of 'fixed air'- a gas which we now call Carbon Dioxide.

Given the fact that in the first experiment the coal had completely disappeared and that fixed air had been produced, whereas in the second experiment only respirable air was produced, Lavoisier concluded that fixed air was composed of respirable air and coal.
However, Lavoisier needed more experimental data to explain the release of respirable air in the second experiment.
For his other experiments on calcination, Lavoisier chose phosphorus as the element burns readily and produces phosphoric acid.
Having placed 1/2 g of phosphorus in an open flask, he weighed the flask and phosphorus together and then placed the flask under a bell jar, partially submerged in a bowl of mercury.
After having lit the phosphorus, Lavoisier noted that the mass of the flask had increased by 0.3g whereas the volume of air in the bell jar had increased by 0.3L, leaving the bell jar with a higher percentage of fixed air than normal air. Therefore it follows that the air in the bell jar was fixed by the phosphorus and that this fixation explains the increase in mass of the flask and its content.
Therefore a certain percentage of the air can be fixed. This particular type of air, respirable air, was released by the mercury oxide in the second experiment. Lavoisier concluded from this that Calcination consisted of the fixation of air as opposed to the release of Phlogiston. It was by questioning the existence of Phlogiston that Lavoisier was able to prove Stahl's theory wrong. He also showed that the air in the atmosphere consisted if at least 2 gases: fixed air and oxygen.
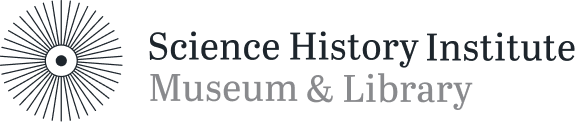

Distillations magazine
Revolutionary instruments: lavoisier’s tools as objets d’art.
In 1788 Antoine-Laurent Lavoisier and Jacques-Louis David were introduced during a sitting for the illustrious scientist’s portrait.
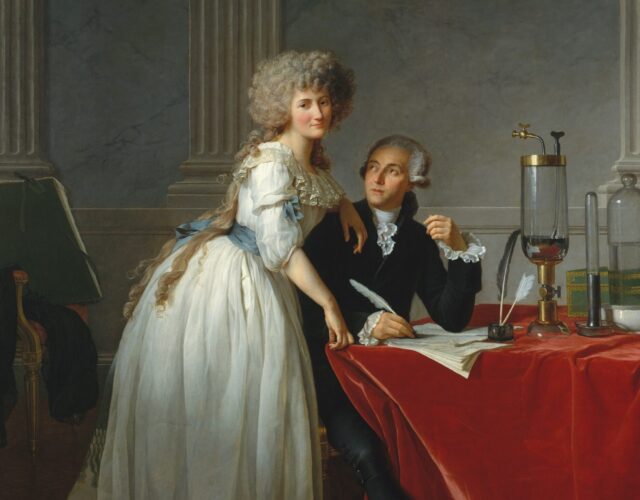
In 1788, just as the stage was set for revolution, France’s most celebrated scientist met with France’s most celebrated artist. This sitting for a portrait of the illustrious scientist and his wife may not have been an entirely cordial meeting. The scientist, Antoine-Laurent Lavoisier (1743–1794), was one of the king’s men; the artist, Jacques-Louis David (1748–1825), would four years later vote for the king’s execution. The rencontre yielded an immense canvas still regarded as one of the greatest portraits of the 18th century.
The meeting in 1788 between Antoine-Laurent Lavoisier (1743–1794) and Jacques-Louis David (1748–1825) resulted in one of David’s finest portraits, an icon of the Enlightenment now hanging in the Metropolitan Museum of Art. The painting shows Lavoisier and his wife and partner in science, Marie Anne Pierrette Paulze (1758–1836). Behind Mme. Lavoisier is a folio, indicating that she is an artist. (As a younger woman, Mme. Lavoisier had studied painting under David’s tutelage, and she may have been the one who instigated the meeting between her husband and her teacher.) In the background of the painting are several pilasters, a signature of David’s neoclassical style. But the most important symbols of Lavoisier’s career are the pieces of chemical equipment. Never mind that they belong in the laboratory and look strangely out of place on a writing desk. They are shown prominently in Lavoisier’s studio so that the viewer knows that this elegant man was a chemist.
It would be interesting to know how the specific pieces of equipment depicted in the portrait were chosen. One can imagine the Lavoisiers showing David their nearly 200 pieces of scientific equipment, many of them beautifully crafted by Nicolas Fortin, Lavoisier’s instrument maker since 1783. Lavoisier and his wife might have chosen pieces for their scientific significance, but David was likely also looking for pieces that would contribute to the overall composition of the portrait. He might also have wanted to paint instruments that would showcase his own skill as a painter at representing reflective surfaces —and this they certainly accomplish. The viewer has no doubt that the glass is glass, the brass is brass, the water is water, and the mercury is mercury.
It may be that the work on Lavoisier’s desk is the manuscript of Traité élémentaire de chimie , which one year after the painting was created would introduce to the world the basic concepts and nomenclature of modern chemistry. The scientific community recognized its importance immediately. Published first in Paris in 1789, it was quickly translated into English as Elements of Chemistry , and Lavoisier became the acknowledged leader of the Chemical Revolution. The popularity of the Traité led to a second edition, published in Paris in 1793, less than a year before Lavoisier stepped up to the guillotine on 8 May 1794. His chemical revolution was well under way as his head and body were carted off to a mass grave.
In the first of the two volumes of the Traité , Lavoisier presents the conclusions and principles derived from his experiments. The second volume describes his experimental methods in detail. Appended to the second volume are 13 plates that show some 170 pieces of laboratory equipment finely drawn to scale by Mme. Lavoisier. Most of these flasks, bottles, jars, siphons, furnaces, tables, and basins do not grace David’s portrait, and those that do are probably the best-known pieces of laboratory glassware in the art world. They also were vital components in several of Lavoisier’s experiments —experiments in which he discovered scientific principles that lie at the very center of modern chemistry.
Mass of Reactants = Mass of Reaction Products
As one might strengthen a rectangular gate with a diagonal brace, David strengthened his rectangular portrait with strong diagonals from the upper left-hand corner to the lower right. Mme. Lavoisier’s right arm, Lavoisier’s quill, the bright fold in the table cover, Lavoisier’s unnaturally long leg, and a beam of light coming from the upper left window all point to a glass balloon on the floor at the lower right of the canvas. The gleaming balloon shows David’s skill to great effect, but it is also important for its use in the establishment of the law of the conservation of mass.
Lavoisier was a superb quantitative chemist, a master of the volumetric flask, the beam balance, the barometer, and the thermometer. Most of his quantitative experiments were performed in closed systems and involved either the consumption or production of gases, which were measured in volumes. In order to balance his equations, the volumes of gases had to be converted to masses. To determine the mass per volume of atmospheric air, nitrogen, oxygen, hydrogen, and carbon dioxide, he weighed the gases in glass balloons, like the one in David’s painting, with capacities of about 17 liters. Each balloon had a brass cap cemented to its neck, through which a metal tube with a stopcock was soldered. Lavoisier measured the balloon’s precise volume by weighing it first empty and again filled with water. He then dried the balloon and evacuated it as much as possible using a brass air pump, visible in the painting. He then closed the stopcock and screwed it to a reaction vessel that contained the gas to be weighed. As the stopcock was opened, the gas rushed into the balloon. Lavoisier then closed the stopcock and weighed the balloon again with, as he writes in the Traité , “the most scrupulous exactitude.” He subtracted the weight of the evacuated balloon and made corrections for temperature, pressure, and incomplete evacuation by the air pump. It is remarkable that the ratios of his measured weights of various gases are not very different from the ratios of their molecular weights, of which Lavoisier had no knowledge. Once established, his volume-to-mass conversion factors would allow him to compare masses of reactants and reaction products.
The law of conservation of mass, which French students call Lavoisier’s law, would soon have enormous repercussions not only for quantitative chemistry but also for understanding the very nature of matter. Lavoisier had shown that regardless of the physical state of the substances involved in a chemical reaction, the total mass of the system must remain unchanged. Such a concept required some number of indestructible particles of constant weight to be present in the reactants and in equal numbers in the reaction products. This led to the atomic hypothesis of the English chemist John Dalton and to the modern understanding of the physical structure of matter.
Water → Hydrogen + Oxygen
The middle instrument on the table is a glass tube about 2 inches in diameter and 24 inches in length, with a flared mouth. This plain and simple device adds to the verticality of the objects on the table, but it also had great meaning for Lavoisier and the Chemical Revolution. With it he was able to show that water was not elemental, but rather that it could be further broken down into hydrogen and oxygen.
Since ancient times water had been considered a basic element. But by 1781 the world was forever changed when water was shown to be, of all things, a combination of two gases. Joseph Priestley, Henry Cavendish, James Watt, and Lavoisier all contributed to that momentous discovery, with Priestley producing water by heating lead oxide in an atmosphere of hydrogen and Cavendish and Watt producing it by burning hydrogen in atmospheric air. All three were so preoccupied with trying to explain their findings in terms of phlogiston theory that it remained for Lavoisier, who in 1783 repeated Cavendish’s earlier experiments, to interpret the reaction correctly: water was being synthesized from hydrogen and oxygen.
But Lavoisier felt that proof of the composition of water was not complete. In the Traité he wrote: “Chemistry affords two general methods of determining the constituent principles of bodies, the method of analysis, and that of synthesis. It ought to be considered as a principle in chemical science, never to rest satisfied without both these species of proofs.” He set out to show the reverse of Cavendish’s synthetic experiment through his own analytic one: the breakdown of water into hydrogen and oxygen.
With the tube completely filled with mercury and inverted in a basin of mercury, as in the portrait, Lavoisier introduced under the lip of the tube small amounts of water and iron filings, both of which floated to the top. The filings gradually lost their metallic luster, and he knew from earlier oxidation experiments that the iron was becoming oxidized, thus removing oxygen from the water. As the iron oxide accumulated on the surface of the mercury, gas collected in the top of the tube. He sampled the gas and found that it burned quietly with a white flame. It was “inflammable air,” which he would later call hydrogen, because it had been “born of water.” Lavoisier considered this the final proof that water is composed of oxygen and hydrogen.
Ethyl Alcohol + Oxygen → Carbon Dioxide + Water
The vessel at Lavoisier’s left hand was suitable for storing oxygen and regulating its release by the stopcock at the top. Surprisingly, it is not included in Mme. Lavoisier’s illustrated inventory, although she did depict functionally similar pieces; it may have been acquired after her plates for the text had been completed. David has given this engaging piece a commanding position on the desk. With its long stem and brass cap, this masterpiece of Nicolas Fortin resembles a giant gold-lipped goblet. There are two stopcocks, one in the stem and one in the metal tube leading out of the airtight brass cap. A long glass tube passes through the cap and down to the bottom of the vessel.
In the Traité Lavoisier described the use of similar vessels. The glass foot of the stem is submerged in a basin of water, and the glass tube is plugged (as it is in the painting). With both stopcocks open, an air pump, screwed to the top stopcock, removes the air from the vessel, causing the water from the basin below to flow into it. Once the vessel is emptied of air and filled with water, the upper stopcock is closed. The glass tube is then attached to an oxygen generator, which produces oxygen by heating either mercuric oxide or red lead oxide. As the oxygen bubbles up through the water, the displaced water exits through the lower stopcock. When sufficient oxygen has accumulated, the glass tube is plugged, the lower stopcock is closed, and the vessel is ready for use as an oxygen storage tank. Certain experiments required a carefully controlled flow of oxygen from the storage tank, and for that the upper stopcock was critical. This is illustrated by an experiment whereby Lavoisier determined the composition of spirit of wine (ethyl alcohol) by combustion.
Lavoisier created a combustion chamber by inverting a bell jar in a basin of mercury and withdrawing part of the air so that the mercury level would rise. He slipped an alcohol lamp containing spirit of wine with “a small morsel” of phosphorus in the wick into the mercury under the lip of the bell jar. It floated to the surface of the mercury, where Lavoisier lit it by quickly pushing a red-hot wire up through the mercury to the lamp and igniting the phosphorus in the lamp’s wick. Thus spirit of wine burned in a closed system of atmospheric air. In pure oxygen the rate of burning would have been explosive, but with the nitrogen of the atmospheric air as a moderator the flame was manageable. As oxygen was consumed by the burning alcohol, water accumulated on the surface of the mercury but the flame grew weaker. To keep the flame going, Lavoisier allowed additional oxygen from the storage vessel, carefully regulated by the upper stopcock, to bubble up through the mercury and into the combustion chamber. In the storage vessel, as oxygen was released via the stopcock, the main chamber filled with the water from its underlying basin by way of the open stopcock in the stem. Too slow a flow of oxygen would extinguish the flame; too rapid a flow would risk overheating and cracking or exploding the bell jar. Lavoisier had learned the hard way that burning alcohol in oxygen in a closed system was hazardous. In his Traité he tells of an instance that “had very near proved fatal to myself, in the presence of some members of the Academy. A violent explosion took place, which threw the jar with great violence against the floor of the laboratory, and dashed it in a thousand pieces.”
When the flame was finally extinguished by the buildup of carbon dioxide in the combustion chamber, Lavoisier closed the stopcock on the oxygen storage tank. Lavoisier had measured the initial weight of the lamp and spirit of wine, as well as the volume of oxygen in the storage tank and the volume of atmospheric air in the combustion vessel. He weighed the water present on the surface of the mercury by withdrawing it with a curved pipette, and after the combustion chamber was dismantled he again weighed the lamp. Before dismantling, Lavoisier measured the volumes of the gases in the system before and after injecting a potassium hydroxide solution into the chamber through the mercury to absorb out the carbon dioxide and by measuring the remaining gas volume, correcting for standard temperature and pressure. The volume of the initial atmospheric air was subtracted, and the gas volumes were converted to weights. The weights of the reactants, alcohol and oxygen, could then be compared with the weights of the products, carbon dioxide and water, to balance the chemical equation.
This and similar procedures with other plant materials led Lavoisier to conclude that “the true constituent elements of vegetables are hydrogen, oxygen, and charcoal [carbon]: These are common to all vegetables, and no vegetable can exist without them.” The door to organic chemistry had been opened. Lavoisier’s experiments showed that the combustion of organic substances resembled animal respiration, consuming oxygen and producing water and carbon dioxide. This bolstered his long-held theory that animal respiration was a form of slow combustion. He would later show that a human being consumes oxygen at a rate proportional to the amount of physical work being done, opening the door to physiological chemistry.
From Portraiture to Politics
At the time the portrait was painted the Chemical Revolution had been firmly established. Now, thanks largely to Lavoisier, balanced chemical equations could be written; heat could be quantified; air and water (considered primordial elements since antiquity) could be broken down into their components; and, as chemical compounds were given compound names, chemistry could be discussed with a new and reasonable nomenclature. Respiration, that mysterious pneuma of the ancients, had become a chemical reaction akin to the burning of an alcohol lamp.
Lavoisier’s instruments are masterfully painted by David, and their realism is astonishing. But as the paint dried, the tools of the Chemical Revolution went back onto laboratory shelves. Lavoisier supervised the government’s gunpowder manufacture and collaborated in collecting taxes for Louis XVI. David, a radical revolutionary, continued his support of the National Convention. The two men went their very separate ways into the political revolution, David as its champion and Lavoisier as its victim.
It would remain for Mme. Lavoisier, the real centerpiece of David’s painting, to promote the contributions that she and her husband had made. After her husband’s death she retrieved the proofs of his unfinished Mémoires de chimie and managed to publish these classic papers, which contained his final interpretations of his work, in 1805. She continued to promote her husband’s discoveries and to be an important figure in the scientific and intellectual life of Paris. But there must have been moments when she longed for those days spent in the laboratory working with her husband, surrounded by the beautiful objets d’art of Nicolas Fortin.
David belonged to a political revolution—Lavoisier to a scientific one. For a brief historical moment these revolutionaries combined their genius to create a work that beautifully captures the brilliance of the social, political, and intellectual upheaval that whirled around them.
Horton A. Johnson is former director of pathology at St. Luke’s–Roosevelt Medical Center, New York City, and professor of pathology at Columbia University College of Physicians and Surgeons. He is also a docent at the Metropolitan Museum of Art.
More from our magazine
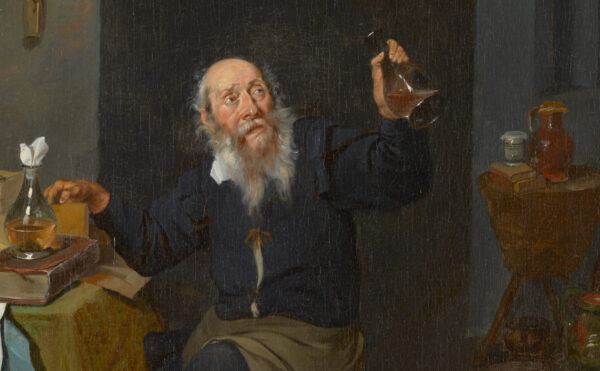
As Good as Gold
Why do we still study the color of urine?
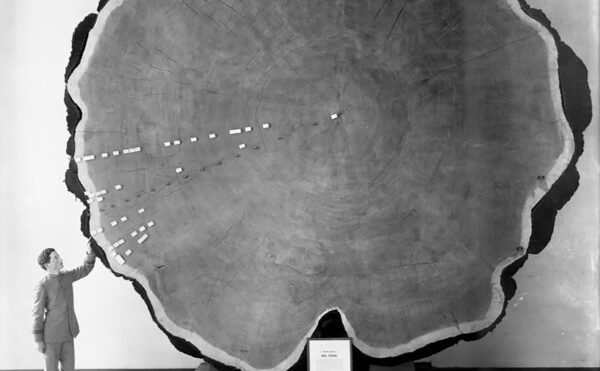
Proxies for Justice
The climate history of tropical regions has been chronically understudied. Correcting the record will require new methods and new mindsets.
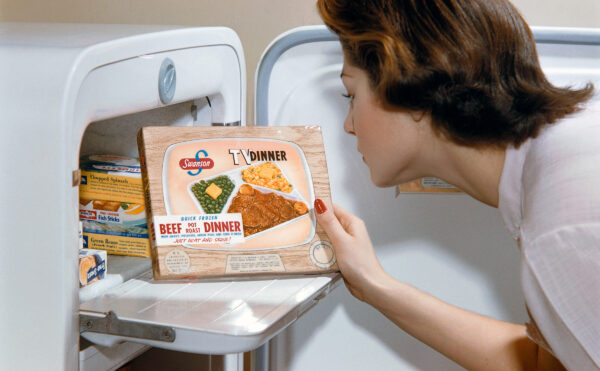
From the Front Line to the Freezer Aisle
How World War II changed the way we eat.
Copy the above HTML to republish this content. We have formatted the material to follow our guidelines, which include our credit requirements. Please review our full list of guidelines for more information. By republishing this content, you agree to our republication requirements.
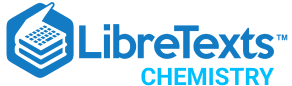
- school Campus Bookshelves
- menu_book Bookshelves
- perm_media Learning Objects
- login Login
- how_to_reg Request Instructor Account
- hub Instructor Commons
Margin Size
- Download Page (PDF)
- Download Full Book (PDF)
- Periodic Table
- Physics Constants
- Scientific Calculator
- Reference & Cite
- Tools expand_more
- Readability
selected template will load here
This action is not available.
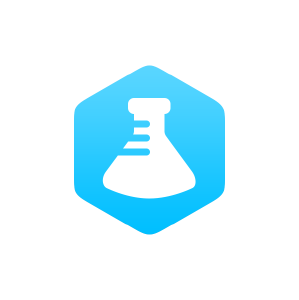
2.3: The Atomic Theory
- Last updated
- Save as PDF
- Page ID 49352
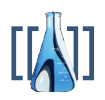
- Ed Vitz, John W. Moore, Justin Shorb, Xavier Prat-Resina, Tim Wendorff, & Adam Hahn
- Chemical Education Digital Library (ChemEd DL)
\( \newcommand{\vecs}[1]{\overset { \scriptstyle \rightharpoonup} {\mathbf{#1}} } \)
\( \newcommand{\vecd}[1]{\overset{-\!-\!\rightharpoonup}{\vphantom{a}\smash {#1}}} \)
\( \newcommand{\id}{\mathrm{id}}\) \( \newcommand{\Span}{\mathrm{span}}\)
( \newcommand{\kernel}{\mathrm{null}\,}\) \( \newcommand{\range}{\mathrm{range}\,}\)
\( \newcommand{\RealPart}{\mathrm{Re}}\) \( \newcommand{\ImaginaryPart}{\mathrm{Im}}\)
\( \newcommand{\Argument}{\mathrm{Arg}}\) \( \newcommand{\norm}[1]{\| #1 \|}\)
\( \newcommand{\inner}[2]{\langle #1, #2 \rangle}\)
\( \newcommand{\Span}{\mathrm{span}}\)
\( \newcommand{\id}{\mathrm{id}}\)
\( \newcommand{\kernel}{\mathrm{null}\,}\)
\( \newcommand{\range}{\mathrm{range}\,}\)
\( \newcommand{\RealPart}{\mathrm{Re}}\)
\( \newcommand{\ImaginaryPart}{\mathrm{Im}}\)
\( \newcommand{\Argument}{\mathrm{Arg}}\)
\( \newcommand{\norm}[1]{\| #1 \|}\)
\( \newcommand{\Span}{\mathrm{span}}\) \( \newcommand{\AA}{\unicode[.8,0]{x212B}}\)
\( \newcommand{\vectorA}[1]{\vec{#1}} % arrow\)
\( \newcommand{\vectorAt}[1]{\vec{\text{#1}}} % arrow\)
\( \newcommand{\vectorB}[1]{\overset { \scriptstyle \rightharpoonup} {\mathbf{#1}} } \)
\( \newcommand{\vectorC}[1]{\textbf{#1}} \)
\( \newcommand{\vectorD}[1]{\overrightarrow{#1}} \)
\( \newcommand{\vectorDt}[1]{\overrightarrow{\text{#1}}} \)
\( \newcommand{\vectE}[1]{\overset{-\!-\!\rightharpoonup}{\vphantom{a}\smash{\mathbf {#1}}}} \)
The development of the atomic theory owes much to the work of two men: Antoine Lavoisier, who did not himself think of matter in terms of atoms but whose work laid organization groundwork for thinking about elements, and John Dalton, to whom the atomic theory is attributed. Much of Lavoisier’s work as a chemist was devoted to the study of combustion. He became convinced that when a substance is burned in air, it combines with some component of the air. Eventually he realized that this component was the dephlogisticated air which had been discovered by Joseph Priestly (1733 to 1804) a few years earlier. Lavoisier renamed this substance oxygen . In an important series of experiments he showed that when mercury is heated in oxygen at a moderate temperature, a red substance, calx of mercury , is obtained. (A calx is the ash left when a substance burns in air.) At a higher temperature this calx decomposes into mercury and oxygen. Lavoisier’s careful experiments also revealed that the combined masses of mercury and oxygen were exactly equal to the mass of calx of mercury. That is, there was no change in mass upon formation or decomposition of the calx. Lavoisier hypothesized that this should be true of all chemical changes, and further experiments showed that he was right. This principle is now called the law of conservation of mass .
As Lavoisier continued his experiments with oxygen, he noticed something else. Although oxygen combined with many other substances, it never behaved as though it were itself a combination of other substances. Lavoisier was able to decompose the red calx into mercury and oxygen, but he could find no way to break down oxygen into two or more new substances. Because of this he suggested that oxygen must be an element —an ultimately simple substance which could not be decomposed by chemical changes.
Lavoisier did not originate the idea that certain substances (elements) were fundamental and all others could be derived from them. This had first been proposed in Greece during the fifth century B.C. by Empedocles, who speculated that all matter consisted of combinations of earth, air, fire, and water. These ideas were further developed and taught by Aristotle and remained influential for 2000 years.
Lavoisier did produce the first table of the elements which contained a large number of substances that modern chemists would agree should be classifies as elements. He published it with the knowledge that further research might succeed decomposing some of the substances listed, thus showing them not to be elements. One of his objectives was to prod his contemporaries into just that kind of research. Sure enough the “earth substances” listed at the bottom were eventually shown to be combinations of certain metals with oxygen. It is also interesting to note that not even Lavoisier could entirely escape from Aristotle’s influence. The second element in his list is Aristotle’s “fire,” which Lavoisier called “caloric,” and which we now call “heat.” Both heat and light, the first two items in the table, are now regarded as forms of energy rather than of matter.
Although his table of elements was incomplete, and even incorrect in some instances, Lavoisier’s work represented a major step forward. By classifying certain substances as elements, he stimulated much additional chemical research and brought order and structure to the subject where none had existed before. His contemporaries accepted his ideas very readily, and he became known as the father of chemistry.
John Dalton (1766 to 1844) was a generation younger than Lavoisier and different from him in almost every respect. Dalton came from a working class family and only attended elementary school. Apart from this, he was entirely self-taught. Even after he became famous, he never aspired beyond a modest bachelor’s existence in which he supported himself by teaching mathematics to private pupils. Dalton made many contributions to science, and he seems not to have realized that his atomic theory was the most important of them. In his “New System of Chemical Philosophy” published in 1808, only the last seven pages out of a total of 168 are devoted to it!
The postulates of the atomic theory are given below. The first is no advance on the ancient Greek philosopher Democritus who had theorized almost 2000 years earlier that matter consists of very small particles.
The Postulates of Dalton's Atomic Theory
- All matter is composed of a very large number of very small particles called atoms.
- For a given element, all atoms are identical in all respects. In particular all atoms of the same element have the same constant mass, while atoms of different elements have different masses.
- The atoms are the units of chemical changes. Chemical reactions involve the combination, separation, or rearrangement of atoms, but atoms are neither created, destroyed, divided into parts, or converted into atoms of any other kind.
- Atoms combine to form molecules in fixed ratios of small whole numbers.
The second postulate, however, shows the mark of an original genius; here Dalton links the idea of atom to the idea of element . Lavoisier’s criterion for an element had been essentially a macroscopic, experimental one. If a substance could not be decomposed chemically, then it was probably an element. By contrast, Dalton defines an element in theoretical, sub-microscopic terms. An element is an element because all its atoms are the same . Different elements have different atoms. There are just as many different kinds of elements as there are different kinds of atoms.
Now look back a moment to the physical states of mercury, where sub-microscopic pictures of solid, liquid, and gaseous mercury were given. Applying Dalton’s second postulate to this figure, you can immediately conclude that mercury is an element, because only one kind of atom appears. Although mercury atoms are drawn as spheres in the figure, it would be more common today to represent them using chemical symbols. The chemical symbol for an element (or an atom of that element) is a one- or two-letter abbreviation of its name. Usually, but not always, the first two letters are used. To complicate matters further, chemical symbols are sometimes derived from a language other than English. For example the symbol for Hg for mercury comes from the first and seventh letters of the element’s Latin name, hydrargyrum.
The chemical symbols for all the currently known elements are listed above in the table, which also includes atomic weights. These symbols are the basic vocabulary of chemistry because the atoms they represent make up all matter. You will see symbols for the more important elements over and over again, and the sooner you know what element they stand for, the easier it will be for you to learn chemistry. These more important element have been indicated in the above table by colored shading around their names.
Dalton’s fourth postulate states that atoms may combine to form molecules. An example of this is provided by bromine, the only element other than mercury which is a liquid at ordinary room temperature (20°C). Macroscopically, bromine consists of dark-colored crystals below –7.2°C and a reddish brown gas above 58.8°C. The liquid is dark red-brown and has a pungent odor similar to the chlorine used in swimming pools. It can cause severe burns on human skin and should not be handled without the protection of rubber gloves.
Figure \(\PageIndex{1}\) : Sub-microscopic view of the diatomic molecules of the element bromine (a) in the gaseous state (above 58°C); (b) in liquid form (between -7.2 and 58.8°C); and (c) in solid form (below -7.2°C).
The sub-microscopic view of bromine in the following figure is in agreement with its designation as an element—only one kind of atom is present. Except at very high temperatures, though, bromine atoms always double up. Whether in solid, liquid, or gas, they go around in pairs. Such a tightly held combination of two or more atoms is called a molecule .
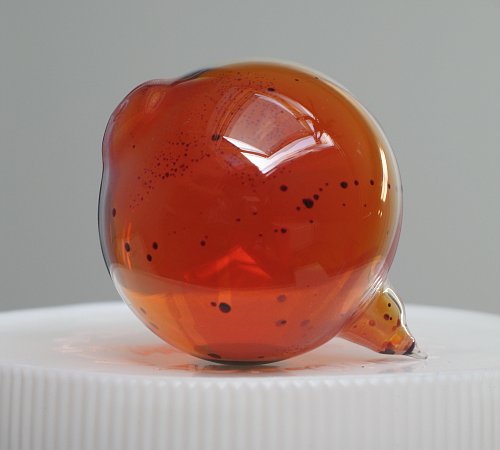
The composition of a molecule is indicated by a chemical formula . A subscript to the right of the symbol for each element tells how many atoms of that element are in the molecule. For example, the atomic weights table gives the chemical symbol Br for bromine, but each molecule contains two bromine atoms, and so the chemical formula is Br 2 . According to Dalton’s fourth postulate, atoms combine in the ratio of small whole numbers, and so the subscripts in a formula should be small whole numbers.
Explain Lavoisier's experiment which provided evidence to the discovery of components in air.
Apparatus : The apparatus was set up as shown, with the mercury in the curved necked retort and air in the bell jar.
Procedure : The mercury in the retort was then heated for several days.
Observation :
- A red layer was formed on the heated mercury surface in the retort.
- The level of mercury in the trough rose by 1 5 \dfrac{1}{5} 5 1 .
Conclusions :
- The oxygen in the retort combined with the mercury forming mercury [II] oxide [red layer] and the level in the trough rose by 1 5 \dfrac{1}{5} 5 1 th of the original volume, thereby occupying the space of the used oxygen in the bell jar.
- The active part of the air removed by mercury on heating was named 'oxygen'.
- The remaining inactive part of the air in the bell jar was then named 'nitrogen'.
Answered By
Related Questions
Name the important scientists and their studies which led to the discovery of the components of air., tabulate the various components of air, including the components with variable composition., compare the main components of air i.e., nitrogen, oxygen and carbon dioxide with reference to their density and solubility, nature and reactivity., state the meaning of the terms — (a) air (b) atmosphere.

Explain Antoine lavoisier experiment on Oxygen
I n experiments with phosphorus and sulfur, both of which burned readily, lavoisier showed that they gained weight by combining with air. with lead calx, he was able to capture a large amount of air that was liberated when the calx was heated. to a suspicious lavoisier, these results were not explained by phlogiston. although lavoisier now realized that combustion actually involved air, the exact composition of air at that time was not clearly understood. in august 1774, the eminent english natural philosopher joseph priestley met with lavoisier in paris. he described how he had recently heated mercury calx (a red powder) and collected a gas in which a candle burned vigorously. priestley believed his "pure air" enhanced respiration and caused candles to burn longer because it was free of phlogiston. for this reason, he called the gas that he obtained from decomposing mercury calx “dephlogisticated air.” in paris, the intrigued lavoisier repeated priestley's experiment with mercury and other metal calces. he eventually concluded that common air was not a simple substance. instead, he argued, there were two components: one that combined with the metal and supported respiration and the other an asphyxiant that did not support either combustion or respiration. by 1777, lavoisier was ready to propose a new theory of combustion that excluded phlogiston. combustion, he said, was the reaction of a metal or an organic substance with that part of common air he termed "eminently respirable." two years later, he announced to the royal academy of sciences in paris that he found that most acids contained this breathable air. lavoisier called it oxygène, from the two greek words for acid generator. lavoisier began his full-scale attack on phlogiston in 1783, claiming that "stahl's phlogiston is imaginary." calling phlogiston "a veritable proteus that changes its form every instant," lavoisier asserted that it was time "to lead chemistry back to a stricter way of thinking" and "to distinguish what is fact and observation from what is system and hypothesis." as a starting point, he offered his theory of combustion, in which oxygen now played the central role..

Which law was given by Antoine L. Lavoisier?
During the experiment done by Antonie Lavoisier for discovery of oxygen, the level of mercury in the bell jar rose by:
Explain an experiment to show that air or oxygen is necessary for combustion. [5 MARKS]

IMAGES
COMMENTS
In Paris, the intrigued Lavoisier repeated Priestley's experiment with mercury and other metal calces. He eventually concluded that common air was not a simple substance. Instead, he argued, there were two components: one that combined with the metal and supported respiration and the other an asphyxiant that did not support either combustion or ...
It was found that the volume was the same as the 16% volume loss. The oxygen percentage (16%) was not accurate, which could be due to not all oxygen react with mercury. From this experiment, we can feel Lavoisier’s emphasize on the conservation of mass in his experiment design.
Nov 1, 2021 · In Paris, the intrigued Lavoisier repeated Priestley’s experiment with mercury and other metal calces. He eventually concluded that common air was not a simple substance. Instead, he argued, there were two components: one that combined with the metal and supported respiration and the other an asphyxiant that did not support either combustion ...
This particular type of air, respirable air, was released by the mercury oxide in the second experiment. Lavoisier concluded from this that Calcination consisted of the fixation of air as opposed to the release of Phlogiston. It was by questioning the existence of Phlogiston that Lavoisier was able to prove Stahl's theory wrong.
Jul 12, 2021 · Lavoisier renamed this substance oxygen. In an important series of experiments he showed that when mercury is heated in oxygen at a moderate temperature, a red substance, calx of mercury, is obtained. (A calx is the ash left when a substance burns in air.) At a higher temperature this calx decomposes into mercury and oxygen.
Apr 7, 2008 · This is illustrated by an experiment whereby Lavoisier determined the composition of spirit of wine (ethyl alcohol) by combustion. Lavoisier created a combustion chamber by inverting a bell jar in a basin of mercury and withdrawing part of the air so that the mercury level would rise.
Jun 16, 2023 · Lavoisier renamed this substance oxygen. In an important series of experiments he showed that when mercury is heated in oxygen at a moderate temperature, a red substance, calx of mercury, is obtained. (A calx is the ash left when a substance burns in air.) At a higher temperature this calx decomposes into mercury and oxygen.
Antoine-Laurent de Lavoisier (/ l ə ˈ v w ɑː z i eɪ / lə-VWAH-zee-ay; [1] [2] [3] French: [ɑ̃twan lɔʁɑ̃ də lavwazje]; 26 August 1743 – 8 May 1794), [4] also Antoine Lavoisier after the French Revolution, was a French nobleman and chemist who was central to the 18th-century chemical revolution and who had a large influence on both the history of chemistry and the history of biology.
The oxygen in the retort combined with the mercury forming mercury [II] oxide [red layer] and the level in the trough rose by 1 5 \dfrac{1}{5} 5 1 th of the original volume, thereby occupying the space of the used oxygen in the bell jar. The active part of the air removed by mercury on heating was named 'oxygen'.
Priestley believed his "pure air" enhanced respiration and caused candles to burn longer because it was free of phlogiston. For this reason, he called the gas that he obtained from decomposing mercury calx “dephlogisticated air.” In Paris, the intrigued Lavoisier repeated Priestley's experiment with mercury and other metal calces.